More than 10 million people a year could die from antibiotic-resistant bacteria, warns a United Nations report. Yet scientists hope that recent advances — from reviving ancient cures to enlisting bacteria-slaying viruses — could prevent that dire prediction.
By the time Tom Patterson, PhD, was medically evacuated from Egypt to Germany after falling ill on vacation, his condition was rapidly deteriorating. Patterson, 68, had become infected with Acinetobacter baumannii, one of the most antibiotic-resistant superbugs known to science. None of the antibiotics German doctors tried could contain the spreading infection. Patterson was finally transported to the University of California, San Diego, (UC San Diego) hospital, where the infection spread to his bloodstream and he lapsed into a coma. Doctors there told his family that he was almost certainly going to die.
Bacteria like Acinetobacter baumannii, resistant to almost every antibiotic in the pharmacological arsenal, are on the rise. When the Centers for Disease Control and Prevention (CDC) issued a landmark report on the issue in 2013, the agency estimated that 2 million Americans contract antibiotic-resistant bacterial infections each year and 23,000 die of them. The toll today is far deadlier. A 2018 study pegged the annual number of deaths in the United States at between 153,113 and 162,044. And in a 2019 report called No Time to Wait, the United Nations warned that by 2050, multidrug resistant infections could kill upwards of 10 million people a year around the world.
“It’s all hands on deck. The problem is so big, and getting bigger, that we need to pursue every promising lead.”
David S. Weiss, PhD
Emory University School of Medicine
To prevent that nightmare from becoming reality, researchers are scrambling to develop new antimicrobials and enhance the power of those we have. Currently, only 16 antibiotics are in development to address difficult-to-treat bacterial threats, according to the Infectious Disease Society of America. And only about 10 to 20% of drugs that enter human trials end up winning approval.
Much more needs to be done, experts warn. “It’s all hands on deck,” says David S. Weiss, PhD, director of the Emory Antibiotic Resistance Center and an associate professor of infectious diseases at Emory University School of Medicine. “The problem is so big, and getting bigger, that we need to pursue every promising lead.”
Here are just a few of the cutting-edge approaches — from unleashing bacteria-destroying viruses to uncovering the secrets of ancient cures — that scientists are pursuing to turn the tide in the race to defeat antibiotic-resistant bacteria.
Phages to the rescue
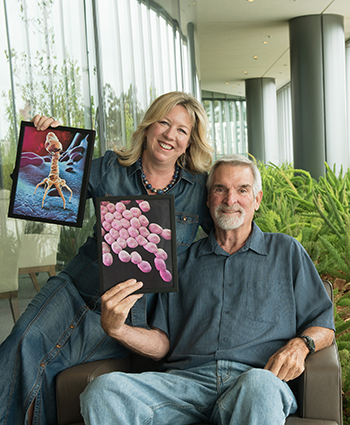
In a case that may mark a turning point in medicine, Tom Patterson didn’t die from his raging infection.
As her husband’s condition grew dire, Steffanie Strathdee, PhD, an infectious disease epidemiologist at UC San Diego, began frantically searching the medical literature for something, anything, that could save him. That’s when she came across an approach that had been used with some success in the 1920s and 30s but had basically been abandoned after penicillin was discovered: using viruses that prey on bacteria, called bacteriophages, to cure bacterial infections.
Bacteriophages — also called phages — attach to bacterial cells and inject genetic instructions, turning their prey into virus-producing factories. In most cases, replicating phages eventually burst the bacterium, destroying it, and proceed to invade other bacterial cells.
Working with Robert Schooley, MD, Patterson’s physician and an infectious disease expert at UC San Diego Health, Strathdee connected with scientists at two institutions engaged in phage research: Texas A&M and the Naval Medical Research Center. Isolates of the superbug running rampant in her husband’s body were sent to both institutions. There, researchers identified eight phage variants that were likely to be active against his infection. Once the hospital and the Food and Drug Administration provided approvals, Schooley administered the experimental phage cocktail.
“Three days later Tom woke up,” recalls Strathdee, who went on to co-write a book with her husband about their ordeal, called The Perfect Predator: A Scientist’s Race to Save Her Husband from a Deadly Superbug. “Within a week or two, he was off all life support.”
In the wake of that unprecedented success — described in the journal Antimicrobial Agents and Chemotherapy — experts at UC San Diego began offering phage therapy to other patients with multidrug resistant infections who had no other treatment options. In June 2018, the UC San Diego School of Medicine opened the Center for Innovative Phage Applications and Therapeutics, the first such center devoted to phage research and therapy in North America. Since Patterson was treated, Schooley and his colleagues have published several additional reports of successful phage treatments.
“Three days later Tom woke up. Within a week or two [of phage therapy], he was off all life support.”
Steffanie Strathdee, PhD
“There’s still a lot we need to learn about dosing, dose frequency, and how to prevent bacteria from becoming resistant to phages,” explains Schooley, who co-directs the center with Strathdee. While acknowledging that such progress will require well-designed clinical trials, Schooley remains confident. “We’re convinced that phage therapy will be an important weapon in our armamentarium against multiresistant infections,” he says.
Small molecules, big promise
Most of today's antibiotics have come from a surprising source: bacteria. In fact, just one type of bacteria — Streptomyces and their cousins — produce two out of three antibiotics now in use. “Bacteria are constantly waging chemical warfare with other microbes, so they’ve evolved all kinds of weapons that we can use,” explains Joshua Blodgett, PhD, a biologist at the University of Washington in St. Louis.
Until recently, researchers assumed that they had already identified most antimicrobial substances Streptomyces produce. But when researchers gained the ability to sequence bacterial genes, they discovered something unexpected. Streptomyces, which scientists thought could produce only three or four drug compounds, contained genetic instructions for as many as 40 small, distinct molecules that could potentially yield medicines.
For reasons that scientists are only beginning to understand, the bacteria don’t actively produce all these molecules. But the hope is that since they contain the necessary genetic instructions, they could be tweaked to make the molecules — something Blodgett’s lab and others around the country are working to achieve. The next step is to test the molecules for antibacterial properties. Then, if they look promising, the molecules will need to be produced in quantities large enough to create medicines.
“There’s still a lot of work to do,” says Blodgett, whose lab recently won a prestigious National Science Foundation grant to study the molecular switches that control antimicrobial molecules. “But we’re excited about the potential here. Streptomyces represent a very small part of the tree of life, one small leaf at the end of one small branch, but they are incredibly endowed with the ability to make things that can kill the bacteria that threaten us. I think they still have a lot to offer us.”
Clues from traditional medicine
Scientists typically turn to the latest journals to guide their research. Ethnobotanist Cassandra Quave, PhD, an assistant professor in Emory University’s Center for the Study of Human Health and in the School of Medicine’s Department of Dermatology, often pores over such ancient sources as 16th-century Chinese herbal texts instead.
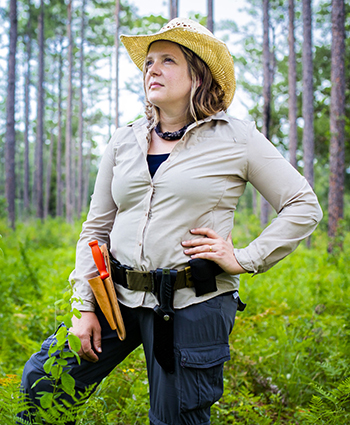
Quave believes that some of the estimated 28,000 plants thought to have medicinal properties may hold keys to addressing the crisis of multidrug resistance.
In addition to consulting ancient texts, Quave interviews local healers in sometimes remote locations as she tracks down traditional, plant-based remedies. She and her team focus on skin infections in part because traditional healers can more easily see the effectiveness of a dermatological remedy than one for a bloodstream infection. And, of course, skin infections can be serious and even life-threatening.
Once Quave identifies a promising natural source, she and her colleagues study its chemical components and their potential bioactivity. The team has already turned up several promising leads, including extracts from the weedy, invasive peppertree.
For centuries, traditional healers in Brazil have used peppertree extracts to treat skin ulcers. In 2017, in results published in Scientific Reports, Quave’s lab captured some of these substances’ promise in defeating bacteria. Unlike standard antibiotics, the extracts don't actually kill bacteria, though. Instead, they can block essential communications between bacteria and prevent them from releasing toxins that cause tissue death. Although the bacteria may survive, they can’t cause as much damage, giving the immune system time to rev up and wipe out the infection.
Another promising candidate is elmleaf blackberry, which has been used in the Mediterranean for centuries to treat boils and abscesses. Quave’s lab has discovered that elmleaf blackberry extract can prevent bacteria from creating biofilms, or thin layers of microbes. “Bacteria need to form biofilms at wound sites in order to better establish infection,” explains Quave. “We’ve shown that combining elmleaf extract and an antibiotic can increase the ability of the antibiotic to eliminate bacteria.”
Quave believes the extract could help remove biofilms that form on medical devices — a dangerous source of hospital-acquired infections. She and her team are also developing bandages coated with the extract that could be used together with conventional antibiotics to eliminate multidrug resistant infections.
“One critique of our approach is a question I often get: Can you really save a patient by targeting the ability of bacteria to communicate or form biofilms?” says Quave. “I do think we can make a real difference. Given the threat of multidrug resistance, we need a multipronged approach, hitting these dangerous pathogens with everything we have.”
The path of partial resistance
Finding new antibiotics is only part of the challenge. Developing better ways to use existing drugs also is critical. At the Emory Antibiotic Resistance Center, Weiss and his team are developing a tool that could be enormously helpful to clinicians faced with multidrug resistant infections.
Today, when clinicians send specimens to the lab for testing, the results indicate only whether bacteria are resistant or susceptible to certain antibiotics. But Weiss’s team has shown that many stubborn infections are partly resistant: some cells are resistant, while others are susceptible. Unfortunately, current lab results may label partially resistant strains as either resistant or as susceptible, making it difficult to identify appropriate treatments. If results indicate that the infection is resistant to a given drug, doctors won’t prescribe it — even though some of the bacteria may be susceptible. And if the results indicate that the strain is susceptible — even when some of the bugs are resistant — doctors may prescribe a single antibiotic that ends up killing only the susceptible cells, leaving the resistant ones to surge back.
The key to treating strains that are partially resistant, says Weiss, is combining two or more antibiotics that work in different ways, dramatically increasing the odds of wiping out resistant bugs. Imagine that a single antibiotic can kill 999 out of 1,000 bacteria. One in 1,000 bacteria will survive. But if doctors also prescribe a second type of antibiotic that can kill 999 out of 1,000 bacteria, the odds of a resistant bug surviving drops to 1 in 1 million.
“It’s critical that we search for new ideas, ones that may be far from our beaten path, if we hope to make progress in a situation that’s fast approaching crisis proportions.”
Ross McKinney, PhD
AAMC
The idea of combining antibiotics to battle stubborn infections isn’t new. But the approach so far has been hit-or-miss. By better understanding partial resistance, Weiss is convinced that even multidrug resistant infections can be defeated with targeted combinations of the antibiotics we have.
In one dramatic example, he and his colleagues tested a highly resistant strain of a superbug called Klebsiella pneumoniae, which doctors isolated from a Nevada woman who was infected while traveling in India. Usually even the most stubborn forms of this infection respond to one of a handful of drugs of last resort. Not this strain. CDC tests showed it was resistant to all 26 drugs that could be used to treat it. The woman went on to die of multiple organ failure and sepsis.
Later, when Weiss’s team studied the strain, they found that the infection was only partially resistant to two drugs. “In the lab, we combined those two drugs and eradicated all the bacteria.”
Armed with that information, doctors might have been able to save the patient. “Our results show that we can identify strains that are partially resistant and then identify specific combinations of antibiotics that will work,” says Weiss. Once the strategy is tested and validated, Weiss hopes that laboratory testing standards will be changed to include partial resistance.
“Clinicians will be able to get the information they need so they don’t prescribe a single antibiotic that isn’t likely to work,” Weiss predicts. “And when they’re up against a really nasty strain and running out of options, the tests will indicate which combinations are most likely to be effective.”
Developing better lab tests and other possible approaches are all worth greater scrutiny, notes Ross McKinney, MD, AAMC chief scientific officer. “The problems of antibiotic resistance are enormous and complex, and sadly not amenable to simple solutions,” he says. "However, it’s critical that we search for new ideas, ones that may be far from our beaten path, if we hope to make progress in a situation that’s fast approaching crisis proportions.”